Materials recovery: The precious prospects within e-waste
This year, the Royal Mint unveiled plans to extract gold from e-waste using a room temperature process, recently developed by the Canadian company Excir (1). For the 2020 Olympic Games in Tokyo, enough gold, silver, and bronze was extracted from two years’ national e-waste to produce all 5000 Olympic medals (2). Could these two separate ventures sufficiently demonstrate the value of e waste to encourage better rates of recycling?
Currently, 50 million tonnes of electronic waste (e-waste) are produced globally every year. Presently, less than 20% of this waste is recycled appropriately, the remainder being incinerated or sent to landfill. The total mass of e-waste production is expected to reach 74 million tonnes by 2030. Unless recycling and reuse rates increase, this will mean more than 59 million tonnes of material wasted, equating to billions of dollars of value (1).
Apart from the “rare” earth elements utilised in the manufacture of electronic devices, particularly batteries, significant quantities of precious metals are present within the circuit boards. All these elements contribute to electronic devices having a high monetary value at the time of disposal. Additionally, the decreasing availability of materials in the earth’s crust and their low concentrations where they are available (average concentrations in ore: copper 50 ppm, silver 0.07 ppm, and gold 0.005 ppm) (3), the ability to extract these materials from end-of-life electronics, where their presence can be measured as a percentage, is becoming increasingly more attractive.
Apart from the “rare” earth elements utilised in the manufacture of electronic devices, particularly batteries, significant quantities of precious metals are present within the circuit boards. All these elements contribute to electronic devices having a high monetary value at the time of disposal. Additionally, the decreasing availability of materials in the earth’s crust and their low concentrations where they are available (average concentrations in ore: copper 50 ppm, silver 0.07 ppm, and gold 0.005 ppm) (3), the ability to extract these materials from end-of-life electronics, where their presence can be measured as a percentage, is becoming increasingly more attractive.
With this consideration, the organisers of the 2020 Tokyo Olympic Games (2) and the British Royal Mint (1, 4), respectively, have made efforts to extract the precious metals from e-waste, whilst the Urban Mining Innovation Centre at the University of British Columbia are looking to develop processes to make mobile phones 100% recyclable.
2020 Tokyo Olympic medals
The Rio de Janeiro 2016 Olympic medals utilised recycled silver, obtained from sources such as mirrors, car parts and x-ray plates and copper (for the bronze medals) came from mint waste (2). This was a significant step for the global sporting celebration towards a sustainable model. This step was not only equalled, but far exceeded by the 2020 Tokyo Olympic Games organisers, who sought to achieve production of the required 5,000 medals from entirely recycled e-waste materials.
In April 2017, the Tokyo Olympic Committee initiated a two-year campaign to collect and recycle sufficient e-waste to recover the materials for the manufacture of the medals for the Games. At the beginning of the campaign, 600 municipalities were involved, this number growing to exceed 1,600 by the end of the project in March 2019 (2). Sites opened across the nation to allow people to donate their electronic devices locally to their homes. The result of the campaign was a staggering 80 tonnes of small electronics such as mobile phones and laptops. However, this is an incredibly small portion of the estimated 2 million tonnes of e-waste produced by Japan annually – suggesting that only 0.002% of Japan’s annual e-waste contributed to the Tokyo Medals Project, but this
was a marked increase in recycling of the devices.
Around 250 mobile phones needed to be processed in order to obtain the 6 g (out of 500 g) of gold for each gold medal. In total, around 32 kg of gold, almost 3,500 kg of silver, and around 2,200 kg of bronze (95% copper, 5% zinc) were recovered from the donated waste small electronic devices (2, 5) (Figure 1).
Whilst this was acknowledged as a significant step in the right direction, Professor Maria Holuszko (of the Urban Mining Innovation Centre at the University of British Columbia) expressed concern that this is insufficient (6). Whilst the metals within e-waste is the valuable portion and simpler to recover, the remaining portion of the device can present significant environmental risk if simply sent to landfill or shredded and incinerated, particularly as this represents the majority portion of the device.
The Rio de Janeiro 2016 Olympic medals utilised recycled silver, obtained from sources such as mirrors, car parts and x-ray plates and copper (for the bronze medals) came from mint waste (2). This was a significant step for the global sporting celebration towards a sustainable model. This step was not only equalled, but far exceeded by the 2020 Tokyo Olympic Games organisers, who sought to achieve production of the required 5,000 medals from entirely recycled e-waste materials.
In April 2017, the Tokyo Olympic Committee initiated a two-year campaign to collect and recycle sufficient e-waste to recover the materials for the manufacture of the medals for the Games. At the beginning of the campaign, 600 municipalities were involved, this number growing to exceed 1,600 by the end of the project in March 2019 (2). Sites opened across the nation to allow people to donate their electronic devices locally to their homes. The result of the campaign was a staggering 80 tonnes of small electronics such as mobile phones and laptops. However, this is an incredibly small portion of the estimated 2 million tonnes of e-waste produced by Japan annually – suggesting that only 0.002% of Japan’s annual e-waste contributed to the Tokyo Medals Project, but this
was a marked increase in recycling of the devices.
Around 250 mobile phones needed to be processed in order to obtain the 6 g (out of 500 g) of gold for each gold medal. In total, around 32 kg of gold, almost 3,500 kg of silver, and around 2,200 kg of bronze (95% copper, 5% zinc) were recovered from the donated waste small electronic devices (2, 5) (Figure 1).
Whilst this was acknowledged as a significant step in the right direction, Professor Maria Holuszko (of the Urban Mining Innovation Centre at the University of British Columbia) expressed concern that this is insufficient (6). Whilst the metals within e-waste is the valuable portion and simpler to recover, the remaining portion of the device can present significant environmental risk if simply sent to landfill or shredded and incinerated, particularly as this represents the majority portion of the device.
Royal Mint e-Waste recycling plant
In March 2022, the British Royal Mint announced that they were constructing a plant within their secure site for the extraction of gold and other precious metals from e-waste (4). The facility will use a process developed by Excir which achieves over 99% recovery of the precious metals at room temperature. The site is expected to be fully operational in 2023.
In March 2022, the British Royal Mint announced that they were constructing a plant within their secure site for the extraction of gold and other precious metals from e-waste (4). The facility will use a process developed by Excir which achieves over 99% recovery of the precious metals at room temperature. The site is expected to be fully operational in 2023.
The average annual gold mining output is over 2,500 tonnes, whilst annual global recovery is approximately 900 tonnes. This includes anode slime, jewellery, dentistry, and electronics scraps, and other material sources. The extraction from gold ores, as well as secondary sources, typically involves the use of cyanide, making the process potentially toxic, to form the water-soluble complex gold cyanide, e.g. K[Au(CN)2] or Na[Au(CN)2]. When present in solutions at a pH less than 11, 99% of the cyanide exists as hydrogen cyanide gas (3). Over a period of 5 hours, this process typically recovers < 5% of the gold in the source material (3).
Thiosulphate has been the most commonly studied alternative to cyanide for gold leaching from ores and other sources (3). Benefits of its use include faster leaching kinetics, lower toxicity and a higher rate of gold recovery in the case of some refractory gold ores. However, the chemistry is complex and requires the use of ammonia, meaning that the process is still toxic, involving high thiosulphate consumption. Thiourea is another well studied gold leaching agent (3). However, the technique using it requires high consumption of thiourea and, presently, no techniques have been developed to recover the gold leached from solution, following this process.
The Excir method’s novelty lies in its use of water-miscible and partially water-miscible organic solvents for the dissolution of gold from ores and secondary sources, whilst saving energy and material (3). The method allows recovery of gold, platinum and palladium from compounds and mixtures using a combination, under appropriate conditions, of an acid, an oxidising agent, and a water miscible or partially water-miscible organic solvent. Their research found that mixtures of hydrochloric acid and hydrogen peroxide or calcium hypochlorite in ethyl acetate or acetonitrile leached greater than 99% of the available gold from a mixed or compound source within a short period of time, at room temperature.
A benefit of this process is that, whilst impurities can be reduced during the gold reduction process, the gold precipitate can be purified by solvent extraction. The solvent can then be evaporated from the leached precious metals and condensed for reuse, reducing the consumption of materials and energy overall. The patent outlines that the process can use a wide range of purification, precipitation, and filtration methods to achieve the desired purity of precious metal product. Additionally, the method allows use of any of a number of compatible acids, oxidising agents, and water-miscible or partially water-miscible solvents.
The Excir process has greater efficiency than cyanide-based processes. This is achieved by the simultaneous leaching and solvent extraction of the precious metals from the source material, whilst the cyanide-based processes achieve these as separate steps. The solvent can also be recovered and reused from the process as developed by Excir, reducing material consumption, combined with reduced time requirements and the ability to complete the process at temperatures between 10°C and 80°C, the energy and material requirements are drastically reduced. The process also provides the fastest known dissolution rate for gold in organic or aqueous systems (6020 gm-2h-1 at room temperature and 9000 gm-2h-1 at 60°C). Less water consumption and safer materials mean that the environmental benefits of this process are not only based in the resourcing of precious metals from secondary sources, rather than mining, but also the reduced impact of the process compared with other methods of leaching gold.
This method will be utilised at the Royal Mint’s new plant from 2023 onwards to process an expected 90 tonnes of e waste per year, producing hundreds of kilograms of gold from this waste (4). The UK produces the second highest quantity of e-waste within Europe, after Norway. Less than 20% of e-waste is currently recycled and, of this, only a small portion is reused. With a current global production of 50 million tonnes of e-waste per year, forecast to rise to 74 million tonnes per year, this process, along with the efforts of the Urban Mining Innovation Centre, could make the proper recycling and material recovery from e-waste more attractive to many industries, resulting in a potential improvement in the rate of these activities.
In the meantime, the Royal Mint’s new facility will support around 40 new jobs and begin to make use of the $57 billion dollars of precious metals contained within e-waste every year.
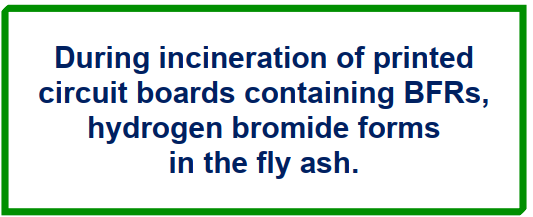
Urban Mining Innovation Centre
Professor Maria Holuszko, of the University of British Columbia (UBC), founded the Urban Mining Innovation Centre within the university. The goal of this centre is to develop techniques and processes which allow recycling and recovery of the entire material make-up of a mobile phone (6).
Professor Holuszko has also collaborated with Professor Clara Satato, of Polytechnic Montreal, in training engineering professionals in Sustainable Electronics and Eco-Design (SEED). This includes training design, manufacture and recycling of electronics. It is also hoped that these engineering professionals will become educated in urban mining and go on to develop economically, environmentally beneficial, safe methods of recovering and repurposing the materials from e-waste, whilst minimising both loss of material and impact to the environment (7).
The team at UBC also made advances toward their goal of 100% recyclability of mobile phones, in 2018, when Professor Holuszko and Amit Kumar developed a process for the separation of fiberglass and resin. This is a massive step towards reducing the waste portion of mobile phones and other electronic devices utilising printed circuit boards – which comprise a much more significant proportion of the device’s mass than precious metals (7).
Professor Maria Holuszko, of the University of British Columbia (UBC), founded the Urban Mining Innovation Centre within the university. The goal of this centre is to develop techniques and processes which allow recycling and recovery of the entire material make-up of a mobile phone (6).
Professor Holuszko has also collaborated with Professor Clara Satato, of Polytechnic Montreal, in training engineering professionals in Sustainable Electronics and Eco-Design (SEED). This includes training design, manufacture and recycling of electronics. It is also hoped that these engineering professionals will become educated in urban mining and go on to develop economically, environmentally beneficial, safe methods of recovering and repurposing the materials from e-waste, whilst minimising both loss of material and impact to the environment (7).
The team at UBC also made advances toward their goal of 100% recyclability of mobile phones, in 2018, when Professor Holuszko and Amit Kumar developed a process for the separation of fiberglass and resin. This is a massive step towards reducing the waste portion of mobile phones and other electronic devices utilising printed circuit boards – which comprise a much more significant proportion of the device’s mass than precious metals (7).
There exist several issues with these components and materials which mean that they are not recycled. Firstly, they are more complex to recover, in that they cannot simply be leached or dissolved and therefore extracted. These materials also have a lower monetary value, and they are more abundant, which means that there is much lower incentive to recover them from end-of-life products (8). Their impact to the environment, however, and the general public’s improving awareness and sensitivity, are key reasons to pursue advances, such as those made by Professor Holuszko and Mr Kumar to, not only develop the processes, but also to scale them up and apply them to real-world waste streams.
Apart from the fiberglass being recovered, this process will limit the addition of brominated flame-retardants (BFRs) into the environment through the disposal of printed circuit boards. BFRs are used to ensure that the circuit boards can operate within a range of temperatures without damaging the components and to reduce the risk of fire within the devices (9).
During incineration of printed circuit boards containing BFRs, hydrogen bromide forms in the fly ash produced, as well as polybrominated dibenzodioxins and dibenzofurans being released to air (10). These present hazards to the environment and, potentially to human health. Hydrogen bromide is harmful, as a gas, at concentrations of 2 ppm and acu tely toxic to humans a t only 30 ppm. Polybrominated dibenzodioxins and dibenzofurans can also enter food sources for ecosystems and studies have revealed them to be highly toxic, causing death in rats within 30 days or potentially bioaccumulating through the food chain (11).
In landfill, BFRs can be leached from the printed circuit boards and volatilisation occurs, meaning easy and rapid uptake into ecosystems, which can be very damaging. These compounds, which are also toxic, have been found in ecosystems, including terrestrial and water-based systems, far from the landfill sites where they originate. These compounds are persistent and bioaccumulate, presenting greater risks at higher levels of the food chain. The distance that these compounds are able to travel is also astonishing, with studies revealing the presence of BFRs in the Arctic. Investigations have revealed that plants and wildlife throughout the natural and cultivated food chains, as well as human tissues, contain BFRs at varying concentrations. Evidence also indicates their potential for various methods of toxicity and endocrine disruption, though little study has been made into their environmental fate and biodegradability.
The method developed by Professor Holuszko and Amit Kumar utilises the different densities of the glass fibres and the resin to achieve gravity separation of the two materials. The separated fiberglass can be used for construction and insulation materials. There is also the potential for its reuse in circuit board fibreglass. However, the reports on the study do not indicate that the resin can be reused, nor its fate following the separation from the fiberglass.
Apart from the fiberglass being recovered, this process will limit the addition of brominated flame-retardants (BFRs) into the environment through the disposal of printed circuit boards. BFRs are used to ensure that the circuit boards can operate within a range of temperatures without damaging the components and to reduce the risk of fire within the devices (9).
During incineration of printed circuit boards containing BFRs, hydrogen bromide forms in the fly ash produced, as well as polybrominated dibenzodioxins and dibenzofurans being released to air (10). These present hazards to the environment and, potentially to human health. Hydrogen bromide is harmful, as a gas, at concentrations of 2 ppm and acu tely toxic to humans a t only 30 ppm. Polybrominated dibenzodioxins and dibenzofurans can also enter food sources for ecosystems and studies have revealed them to be highly toxic, causing death in rats within 30 days or potentially bioaccumulating through the food chain (11).
In landfill, BFRs can be leached from the printed circuit boards and volatilisation occurs, meaning easy and rapid uptake into ecosystems, which can be very damaging. These compounds, which are also toxic, have been found in ecosystems, including terrestrial and water-based systems, far from the landfill sites where they originate. These compounds are persistent and bioaccumulate, presenting greater risks at higher levels of the food chain. The distance that these compounds are able to travel is also astonishing, with studies revealing the presence of BFRs in the Arctic. Investigations have revealed that plants and wildlife throughout the natural and cultivated food chains, as well as human tissues, contain BFRs at varying concentrations. Evidence also indicates their potential for various methods of toxicity and endocrine disruption, though little study has been made into their environmental fate and biodegradability.
The method developed by Professor Holuszko and Amit Kumar utilises the different densities of the glass fibres and the resin to achieve gravity separation of the two materials. The separated fiberglass can be used for construction and insulation materials. There is also the potential for its reuse in circuit board fibreglass. However, the reports on the study do not indicate that the resin can be reused, nor its fate following the separation from the fiberglass.
Conclusion
There are many directives driving improvements in the reuse and recycling of waste electronics and electrical equipment. Among these are the Waste Electronic and Electrical Equipment Directive (WEEE, 2012), Restriction of the use of certain Hazardous Substances Directive (RoHS 2011/65/EU), Energy Related Products Directive (ErP 2009/125/EC) and the Energy-using Products Eco-Design Directive (EuP). These place the onus and cost of recycling and disposal of waste electronics on the original manufacturer. Furthermore, they require improvements in the energy efficiency and useable lifetime of consumer electronics, in order to reduce the rate of waste (8).
However, whilst these directives have driven improvements, the primary incentives have come from consumer pressure, increasing scarcity of materials, and increasing value of materials that can be recovered. As a result, the direction of manufacturers has been to recover the precious and “rare” earth metals which have high sourcing costs and, therefore, high value. These make up an incredibly small portion of electronic devices, meaning that recovery of the bulk of material comprising the devices must be developed by those whose primary interest is in protecting the environment and limited resources that it has to offer.
Whilst the Tokyo Medals Project and the Royal Mint’s new plant are significant steps in the right direction, they are still only a few paces on a very long route towards sustainable electronics and sufficient recovery of the materials at the time of disposal. This route relies not only on the manufacturers’ attitudes towards design and
material sourcing, but also on consumer and retailer attitudes evolving and becoming more responsible and sustainable to compliment these new techniques. The concern around this is that potentially the largest obstacle to a sustainable electronics economy lies in retailers. Electronics retailers have no legal nor financial responsibility for safe and sustainable manufacture, use or disposal, however, they stand to make the greatest profit from rapid product turnover and replacement.
There are many directives driving improvements in the reuse and recycling of waste electronics and electrical equipment. Among these are the Waste Electronic and Electrical Equipment Directive (WEEE, 2012), Restriction of the use of certain Hazardous Substances Directive (RoHS 2011/65/EU), Energy Related Products Directive (ErP 2009/125/EC) and the Energy-using Products Eco-Design Directive (EuP). These place the onus and cost of recycling and disposal of waste electronics on the original manufacturer. Furthermore, they require improvements in the energy efficiency and useable lifetime of consumer electronics, in order to reduce the rate of waste (8).
However, whilst these directives have driven improvements, the primary incentives have come from consumer pressure, increasing scarcity of materials, and increasing value of materials that can be recovered. As a result, the direction of manufacturers has been to recover the precious and “rare” earth metals which have high sourcing costs and, therefore, high value. These make up an incredibly small portion of electronic devices, meaning that recovery of the bulk of material comprising the devices must be developed by those whose primary interest is in protecting the environment and limited resources that it has to offer.
Whilst the Tokyo Medals Project and the Royal Mint’s new plant are significant steps in the right direction, they are still only a few paces on a very long route towards sustainable electronics and sufficient recovery of the materials at the time of disposal. This route relies not only on the manufacturers’ attitudes towards design and
material sourcing, but also on consumer and retailer attitudes evolving and becoming more responsible and sustainable to compliment these new techniques. The concern around this is that potentially the largest obstacle to a sustainable electronics economy lies in retailers. Electronics retailers have no legal nor financial responsibility for safe and sustainable manufacture, use or disposal, however, they stand to make the greatest profit from rapid product turnover and replacement.
References
1. Malloy, K. Royal Mint trials machinery that extracts precious metals from e-waste. Resource, 20 October 2021. https://resource.co/article/royal-mint-trials machinery-extracts-precious-metals-e-waste (Accessed 12.01.2023)
2. Marshall, J. Tokyo 2020 medals made from old phones, laptops. dw.com, 23 July 2021. https://www.dw.com/ en/tokyo-2020-olympic-medals-made-from-old smartphones-laptops/a-58325432 (Accessed 12.01.2023)
3. Foley, S., et al. (2018) United States Patent Application Publication No.: US 2018/0112289 A1. [For more on gold geochemistry and extraction, see: R. W. Boyle, Gold: History and Genesis of Deposits, Springer, New York 1987; ISBN 978-0-442-21162-2, p 11]
4. E&T editorial staff. Royal Mint announces new recycling plant from e-waste. E&T-IET, 21 March 2022. https://eandt.theiet.org/content/articles/2022/03/royal-mint announces-new-recycling-plant-to-extract-gold-from-e waste/ (Accessed 12.01.2023)
5. Brunning, A. What are the Tokyo 2020 Olympicc medals made of? Compoundchem.com, 27 July 2021 https://www.compoundchem.com/2021/07/27/tokyo2020/ (Accessed 12.01.2023)
6. The University of British Columbia. The Urban Mining Innovation Centre. https://mining.ubc.ca/research group/urban-mining-innovation/ (Accessed 12.01.2023)
7. The University of British Columbia. Recycling experts hit milestone in quest for zero waste phone. UBC News, April 12 2018 https://news.ubc.ca/2018/04/12/ recycling-experts-hit-milestone-in-quest-for-zero waste-phone/ (Accessed 12.01.2023)
8. Eduljee, G.H., Harrison, R.M. eds). Electronic Waste Management, 2nd edn. (Issues in Environmental Science and Technology No. 49), Royal Society of Chemistry, Cambridge, 2020; Print ISBN : 978-1-78801-744-2.
9. Mennear, J.H, Lee, C.-C. (1994). Polybrominated Dibenzo-p-Dioxins and Dibenzofurans: Literature Review and Health Assessment. Environmental Health Perspectives, 102(1), 265-274. http://www.jstor.org/stable/4640448
10. Five Winds International, LP For Environment Canada. An Environmental Scan of Toxic and Hazardous Materials in IT and Telecom Products and Waste. Report, 2001.
11. Simonsen, F.A., et al. (2000). Brominated flame retardants: toxicity and ecotoxicity. Danish Environmental Protection Agency, Project, (568).
1. Malloy, K. Royal Mint trials machinery that extracts precious metals from e-waste. Resource, 20 October 2021. https://resource.co/article/royal-mint-trials machinery-extracts-precious-metals-e-waste (Accessed 12.01.2023)
2. Marshall, J. Tokyo 2020 medals made from old phones, laptops. dw.com, 23 July 2021. https://www.dw.com/ en/tokyo-2020-olympic-medals-made-from-old smartphones-laptops/a-58325432 (Accessed 12.01.2023)
3. Foley, S., et al. (2018) United States Patent Application Publication No.: US 2018/0112289 A1. [For more on gold geochemistry and extraction, see: R. W. Boyle, Gold: History and Genesis of Deposits, Springer, New York 1987; ISBN 978-0-442-21162-2, p 11]
4. E&T editorial staff. Royal Mint announces new recycling plant from e-waste. E&T-IET, 21 March 2022. https://eandt.theiet.org/content/articles/2022/03/royal-mint announces-new-recycling-plant-to-extract-gold-from-e waste/ (Accessed 12.01.2023)
5. Brunning, A. What are the Tokyo 2020 Olympicc medals made of? Compoundchem.com, 27 July 2021 https://www.compoundchem.com/2021/07/27/tokyo2020/ (Accessed 12.01.2023)
6. The University of British Columbia. The Urban Mining Innovation Centre. https://mining.ubc.ca/research group/urban-mining-innovation/ (Accessed 12.01.2023)
7. The University of British Columbia. Recycling experts hit milestone in quest for zero waste phone. UBC News, April 12 2018 https://news.ubc.ca/2018/04/12/ recycling-experts-hit-milestone-in-quest-for-zero waste-phone/ (Accessed 12.01.2023)
8. Eduljee, G.H., Harrison, R.M. eds). Electronic Waste Management, 2nd edn. (Issues in Environmental Science and Technology No. 49), Royal Society of Chemistry, Cambridge, 2020; Print ISBN : 978-1-78801-744-2.
9. Mennear, J.H, Lee, C.-C. (1994). Polybrominated Dibenzo-p-Dioxins and Dibenzofurans: Literature Review and Health Assessment. Environmental Health Perspectives, 102(1), 265-274. http://www.jstor.org/stable/4640448
10. Five Winds International, LP For Environment Canada. An Environmental Scan of Toxic and Hazardous Materials in IT and Telecom Products and Waste. Report, 2001.
11. Simonsen, F.A., et al. (2000). Brominated flame retardants: toxicity and ecotoxicity. Danish Environmental Protection Agency, Project, (568).